Fermentation and harvesting scale down for rapid and rational bioprocesses scaling-up
In the bioprocessing industry, fermentation is a unit operation in which microorganisms are grown so as to generate products.
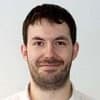
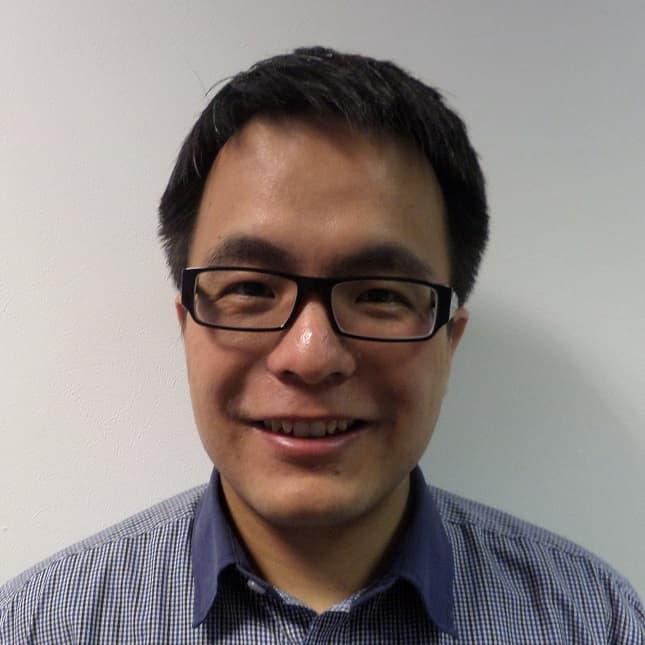
Alex Smith, CEng and Qiang Li, CEng
In the bioprocessing industry, fermentation is a unit operation in which microorganisms are grown so as to generate products.
Fermentation is a mature industry with new challenges
Stirred tanks with turbine impellers are the most widely used type of fermenters for mid-scale industrial fermentation processes, owing to their excellent liquid mixing and gas dispersion capacity. However, scaling-up in such vessels results in varying hydrodynamic environments across different scales, limiting large scale biological production abilities when compared to smaller vessels. At the end of a fermentation, cells are harvested by solid-liquid separation, typically involving filtration and centrifugation. Robust and economical, centrifugation is the preferred option at large scale. Centrifugal separators typically run at high speed and in a high shear environment. A continuous-flow bowl centrifuge with multiple separating discs provides enough solid-liquid separate efficiency and throughput, although scaling-up would be a challenge as it deviates significantly from the typical laboratory centrifuges that run in batch mode and in a low shear environment.
Scale down modelling — Why and how we do it
At CPI we develop and validate tools and methods that apply at small scale to mimic and predict large scale operations. This approach requires only a few millilitres of biomaterial, reducing the scale by a factor of 1 million compared to the typical pilot/production scales used in the industrial biotechnology sector. This allows for a wider window of operations and bioprocessing solutions to be examined under scale-relevant conditions during early stage process development. With this approach, there is the potential to inform, de-risk and de-bottleneck pilot and production scale operations, at the lowest possible cost and at the earliest opportunity. With the full benefits of validated scale-down models, the bioprocessing industry could move their products from proof-of-concept to full commercialisation with greatly enhanced speed, precision and safety.
Applying scale-down methodology to upstream fermentation operations
With regards to fermentations, various scale-down and scale-up strategies and criteria exist in the public domain. Matching stirrer impeller speed, mass transfer rate and stirrer power draw are among those techniques commonly used. At CPI we have found that targeting equal mass transfer rates in geometrically similar stirred tanks gives the most satisfactory results when comparing fermentation performance across multiple scales. We first characterised the reactor geometry and mass transfer coefficient of all our stirred tank fermenters in our National Industrial Biotechnology facilities and development laboratories, ranging from 1L to 10kL scales. We identified the reactor aspect ratio (height to diameter) and impeller spacing as the two parameters most critical to conserve for scalability. Based on this, we chose a 5L stirred tank as the scale down fermenter to mimic pilot scale fermenters at 750L and 10kL scales. We mapped out the maximum theoretical mass transfer rate of those fermenters to the 5L fermenter and identified the operation windows through experiments and computer simulations. Other Critical Process Parameters (CPP) such as temperature, pH and dissolved oxygen were conserved across the scales. Substrate feed rate and airflow rate were normalised to the respective fermenter working volumes and scaled down linearly. With this method, we have shown that the 5L fermenter is able to match performance in cell growth (OD600), substrate (Glucose) utilisation, and inhibitory by-product (Acetic acid) formation with a recombinant E. coli fermentation, when compared against respective process, runs at 750L and 10kL scales. A protein gel image showed similar recombinant protein production in those runs, too.


Scale down of downstream product isolation
Similar to upstream operations, the same scale-down approach is used at CPI to develop and validate scalable process routes for product isolation and purification. Centrifugation is one of the more challenging unit operations to apply scale-down theory, but we routinely apply an in-house methodology for this operation. Regardless of scales and operation modes, biological particles’ sedimentation velocity in any centrifuge conforms to Stokes’ law, and the percentage of the particles escaping sedimentation is determined by the equivalent settling area of any given centrifuge [Qiang et al. 2019)]. As such, we applied this theory to develop the scale-down model below, where Σ is the equivalent settling area of a centrifuge


VLab and tLab are the process volume and time in small scale centrifuges, and QI is the feed flow rate in industrial centrifuges. This scale-down model was tested during the harvesting step of a high-cell-density E. coli fermentation. Two lab-scale centrifuges (at 1mL and 50mL scales) were proven to match the clarification efficiency of two industrial centrifuges, Alfa Laval and Westfalia, in the full process throughput ranging from 120L/h to 8000L/h. Hereby we are able to mimic and predict the large scale centrifugations from small scale bench top centrifuges. This scale down model enables us to understand the scaling-up effect at the harvest step, and to predict and optimise large scale operation at small scale, where time and cost are significantly lower.
When physics augments biology
We also developed a series of Computational Fluid Dynamics (CFD) models to complement and augment our scale-down models. CFD combines the fermenters’ designs with a series of fluid dynamics governing equations (mainly mass and energy balance and turbulence kinetics) to arrive at a numerical solution through complex calculations performed in the background by specialised CFD software packages. With wet-lab experiments to provide data points to hook onto CFD models for calibration and validation purposes, modern CFD models have unparalleled power in simulating and predicting process variates that aren’t readily available or too expensive to measure at scale. So far, this iterative approach has enabled us to simulate and predict turbulence kinetic energy, mixing time and mass transfer coefficient in all of our fermenters with reasonable accuracy. The below graph shows an example CFD simulation of mixing performance in our 10kL fermenter, simulating the mixing of a tracer into the fluid while stirring. The mixing performance is compared here against experimental data and it can be seen that computer simulation matches experiment data well.
On top of mixing CFD models the ability to create realistic and predictive CFD models on mass transfer between two phases for scale-down broadens our horizons and gives us additional insight into the inherent performance of our fermenters. The plots below show a simulation of fluid hydrodynamics disparity between the 10kL and 20L fermenters when air is sparged into the fermenters from the base. It is therefore clear from the results below that the gas holdup and mass transfer at the 10kL scale appears much more uniformly distributed than at the 20L scale. Other observations have also been made through this type of modelling, such as an overall increase in bubble size and gas holdup with increasing vessel scale.


CFD capability empowers us to scale out our processes to a customer’s process, and vice versa. The quantitative difference in the hydrodynamic environment, as revealed by CFD simulations between our own stirred tank fermenters when compared to other fermenters, helps us to design further tests under scaled down conditions to mitigate the risks of large scale operations.
Closing remarks and future perspective
Validated scale down models to mimic and predict large scale operation are useful tools to mitigate the risk of piloting and subsequently demonstrate bioprocesses in a much more efficient and cost-effective way. At CPI we have developed and validated scale down models for upstream bioprocessing steps, fermentation and harvesting. We aim to apply such tools to help customers demonstrate and troubleshoot their bioprocesses at scale. With the advent and the help of other scale-up tools, such as CFD simulation, process modelling and comparative omics, we are able to continue to serve and contribute to the bioprocessing industry in the UK for the future.
References
Qiang Li, Chris Reeve , Lorena Martinez and Stephen Wright. 2019. Scale up biotechnology in Comprehensive biotechnology. 3rd Ed. In press.
Enjoyed this article? Keep reading more expert insights...
CPI ensures that great inventions gets the best opportunity to become a successfully marketed product or process. We provide industry-relevant expertise and assets, supporting proof of concept and scale up services for the development of your innovative products and processes.